Hydrogeology of Sand and Gravel Aquifers in the Owasco Inlet Watershed, Cayuga and Tompkins Counties, New York
Links
- Document: Report (15.9 MB pdf) , HTML , XML
- Plate: Plate 1 (368 MB pdf) - Hydrogeology of the Owasco Inlet Watershed, Cayuga and Tompkins Counties, New York [layered pdf; to toggle layers, download the file (right-click and select "Save link as...") and open it with Adobe Acrobat Reader]
- Figure: Figure 4 (1.03 MB pdf) - Longitudinal hydrogeologic sections A1–A1’ and A2–A2’, spanning the length of the Owasco Inlet valley, Tompkins and Cayuga Counties, New York
- Data Releases:
- USGS data release - Geospatial datasets of the glacial geology and hydrogeology of the Owasco Inlet Watershed, Cayuga and Tompkins Counties, New York
- USGS data release - Horizontal-to-vertical spectral ratio (HVSR) soundings and depth-to-bedrock data for the Owasco Inlet watershed, Cayuga and Tompkins Counties, New York 2016
- Download citation as: RIS | Dublin Core
Acknowledgments
The following town and village officials provided water-supply information and facilitated access to sites for seismic measurements: Chad Shurtleff, Village of Groton, Department of Public Works Supervisor; Peter Compton, Town of Locke, Highway Superintendent, and head of water district maintenance; and Anne Riddell, Village of Moravia, clerk. Municipal water-supply information was provided by Eileen O’Connor, Cayuga County Health Department, Director of Environmental Health Division; and Stephen Maybee, Tompkins County Department of Health, public health engineer. Douglas Freehafer, U.S. Geological Survey (USGS), processed soils and lidar data layers for ARCMAP. Michael Izdebski and Jonathan Stander, USGS volunteers, verified well-permit locations from the New York State Department of Environmental Conservation’s well permit program.
Abstract
This study is a continuation of a series of hydrogeologic appraisals that have been conducted since 1980, as part of a cooperative, long-term, detailed aquifer mapping program by the U.S. Geological Survey and the New York State Department of Environmental Conservation. These appraisals provide a foundation for wellhead protection programs, water-resource management and planning decisions, and groundwater remediation in upstate New York. The Owasco Inlet watershed drains north directly to Owasco Lake, one of New York’s Finger Lakes. The watershed is similar in form to other watersheds of the Finger Lakes region of New York State and is characterized by broad, smooth uplands punctuated by the 18-mile-long Owasco Inlet valley and the secondary valleys of Decker, Dresserville, and Hemlock Creeks. All these streams occupy “through valleys”—the valleys are continuous into adjacent watersheds such that watershed divides are high points within the valley bottoms, rather than in uplands.
Most glacial deposits in the watershed were deposited during the Valley Heads Readvance and subsequent retreat (with at least one minor readvance). Estimates are that the Valley Heads Readvance likely peaked in the Cayuga basin about 17,000 (calendar) years ago based on dates from western New York and the Mohawk Valley in east-central New York. It was the last major ice advance of the Pleistocene Epoch in this region. This readvance covered the entire Owasco watershed.
Valley Heads ice in the Owasco watershed was part of the Cayuga ice lobe, which flowed parallel to the Owasco Inlet valley as far south as Moravia, but which became progressively more eastward south of Locke, effectively raking across the valley from the west. The Owasco Inlet watershed drains north, like other Finger Lakes watersheds, and during deglaciation, northward meltwater drainage was largely blocked by the ice, which resulted in development of a series of regional proglacial lakes in which fine lacustrine sediments predominated. Lacustrine sediments constitute much of the Owasco Inlet valley fill, but it has been pointed out that there are no obvious outlet channels exiting the Owasco Inlet watershed, and it has been proposed that there was northward drainage of meltwater into the ice within the larger, neighboring Cayuga Trough. The lacustrine deposits in the Owasco Inlet valley are commonly underlain by thinner coarse-grained stratified deposits (subaqueous fans and eskers) of variable sorting and permeability. An exception occurs at Groton, N.Y., where retreating ice paused long enough for coarse-grained sediments to fill much of the valley. Smaller, higher elevation valleys have a wider variety of glacial valley-fill deposits, ranging from fine lacustrine sediments to sand and gravel to till.
Groundwater is the sole source of water supply in the area; glacial sand and gravel aquifers are the primary water source in the Owasco Inlet and Decker Creek valleys, and fractured bedrock aquifers typically supply domestic wells in the remaining valleys and upland areas.
Municipal supplies tap a variety of aquifer types in the Owasco Inlet valley and nearby uplands. The hamlet of Locke taps the extensive confined aquifer beneath fine-grained lacustrine deposits in the valley. The Village of Moravia taps a semiconfined aquifer that overlies proglacial lake deposits and is partly confined by overlying recent lake deposits and alluvium. Withdrawals from this aquifer may also induce water from the Owasco Inlet into the aquifer. The Village of Groton draws from two aquifers: (1) a thin upland unconfined sand and gravel aquifer tapped by an infiltration gallery and (2) the local unconfined or semiconfined aquifer in the Owasco Inlet valley. Another aquifer with potential for municipal supply is the unconfined aquifer in the Decker Creek valley near Wilson Corners. The confined aquifer in the lower Dresserville Creek valley may have some water-resource potential, but it is largely untested.
Unconfined aquifers are the most susceptible to contamination from activities at land surface directly above the aquifer because precipitation and subsequent recharge can transport contaminants directly to the water table. Adjacent upland areas can also contribute contaminants. Confined aquifers are less susceptible to contamination from overlying land surface areas because confining units largely prevent downward movement of water. Recharge occurs elsewhere at unconfined upvalley locations and along valley walls where alluvial fans, ice-contact deposits, or stream incision into the valley wall may provide pathways for downward movement of groundwater.
Introduction
This study is a continuation of a series of hydrogeologic appraisals that have been conducted since 1980 in cooperation with the New York State Department of Environmental Conservation (NYSDEC). These reports provide a foundation for wellhead protection programs, water-resource management and planning decisions, and groundwater remediation in upstate New York. In 2015, the U.S. Geological Survey (USGS), in cooperation with the NYSDEC, began an appraisal of the sand and gravel aquifers within the Owasco Inlet watershed, which covers about 117 square miles (mi2) and drains north directly to Owasco Lake (fig. 1), one of New York’s Finger Lakes. About three-quarters of the watershed is within Cayuga County; the remaining area (southern end) is in Tompkins County. Cayuga County towns wholly or partly within the study area include Moravia, Locke, Sempronius, Summerhill, Venice, Genoa, and Niles. Tompkins County towns wholly or partly within the study area include Groton, Dryden, and Lansing. The main villages within the watershed are in the Owasco Inlet valley. From north to south, they include Moravia, Locke, and Groton. Freeville is just south of the watershed boundary.
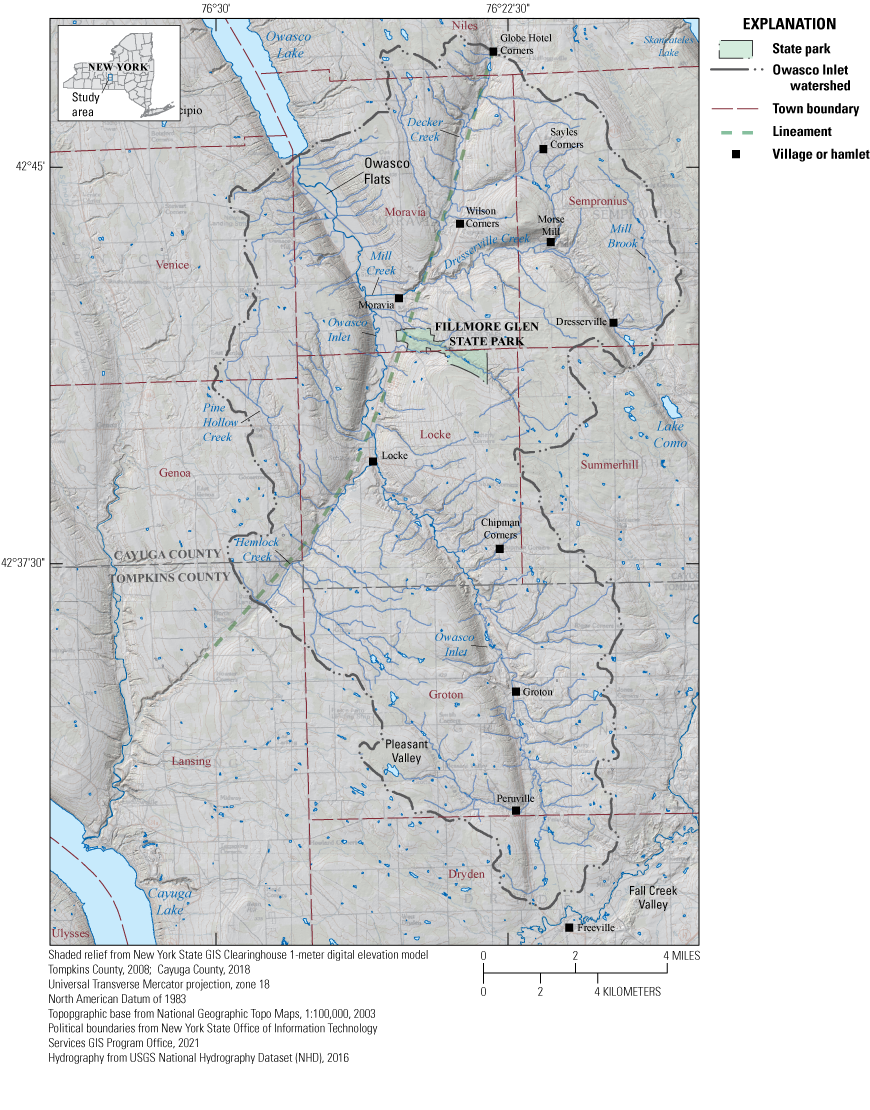
Map showing surface water, place names, political boundaries, and a lineament, Owasco Inlet watershed study area, Cayuga and Tompkins Counties, New York.
The topography of the area is characterized by broad, undissected uplands cut by deep Finger Lakes valleys and some secondary valleys. The Owasco Inlet valley stretches about 18 miles (mi) from Owasco Lake south to the divide at Freeville (fig. 1). Major tributaries include Hemlock Creek on the west side of the watershed and Dresserville and Decker Creeks on the east side. Owasco Inlet and these three tributaries occupy “through valleys,” which means that the valleys are continuous into adjacent watersheds such that the drainage divide is a highpoint of glacial or postglacial valley-fill sediments on the valley floor rather than an upland bedrock ridge. Through valleys are the result of erosion from multiple glaciations.
The Owasco Inlet valley from the lake to Moravia is similar in orientation (southeast) and width to that of Owasco Lake—broad, flat bottomed, and steep sided (fig. 1). From Moravia to Locke, the valley narrows and the orientation shifts from southeast to south-southwest. This segment forms a northeast-southwest-trending arcuate lineament of bedrock weakness along with the valleys of Decker Creek and Hemlock Creek (fig. 1). The Owasco Inlet valley from Locke to just north of Groton is generally symmetrical and oriented southeast with a narrow floodplain. From north of Groton south to Peruville, the valley becomes asymmetrical with a steep wall on the west side and a gradual rise to the divide on the east side; the floodplain is narrow. From Peruville to Freeville, the asymmetry is maintained but the valley widens into a poorly drained basin, broadly open to the south at the junction with the Fall Creek valley. The valley of Dresserville Creek at Moravia is oriented perpendicular to the inlet valley for about 3 mi, but at Morse Mill, the valley turns 90 degrees to the southeast, which is parallel to the inlet valley (fig. 1) from Moravia to Owasco Lake. This indicates an inherent weakness in the bedrock structure that has been accentuated by parallel ice movement and erosion.
Bedrock underlying the study area is mostly shale with some limestone of the Middle to Late Devonian Genesee Group. Older Middle Devonian rocks are exposed on valley walls or subcrop in the Owasco Inlet valley just north of Locke hamlet northward to Owasco Lake. They include the late Middle Devonian Tully Limestone, and the underlying shales and limestones of the Middle Devonian Hamilton Group (Rickard and Fisher, 1970). These rocks are gently folded and dip 20 to 50 feet (ft) per mile to the south.
Purpose and Scope
This report summarizes the hydrogeology of sand and gravel aquifers (primarily the valley-fill aquifer system) in the 117-mi2 Owasco Inlet watershed in Tompkins and Cayuga Counties, New York (fig. 1). An interactive map plate was constructed with layers of information:
-
• Surficial geology
-
• Extent and types of sand and gravel aquifers
-
• Glacially streamlined features (mega flutes), identified from light detecting and ranging (lidar) imagery
-
• Location of geologic sections and links to accompanying illustrations
-
• Location of wells, test borings, and horizontal-to-vertical spectral ratio (HVSR) seismic soundings
-
• Sand and gravel pits, and quarries
-
• Ice-margin positions as defined by moraines and ice-contact deposits
-
• Base map—Built from high-resolution, shaded-relief lidar imagery and 1:24,000-scale USGS topographic maps.
Figures depict the study area extent, upland areas of thick glacial deposits, moraines and other glacial landforms, hydrogeologic sections, sand and gravel aquifer type and distribution within the Owasco Inlet watershed, and a conceptual block diagram of groundwater flow within the hydrogeologic framework. This report summarizes the glacial geology, hydrogeologic framework of glacial deposits within the valley areas, sand and gravel aquifer types and distribution, and municipal groundwater resource use as of 2017. Two data releases accompany the report: the first includes geospatial data used in the map plate and map figures (Heisig, 2023a) and the second includes the HVSR seismic data (Heisig, 2023b).
Previous Work
Ice retreat and surficial geology within the study area have been interpreted in detail by Carney (1909) in his Moravia 15-minute quadrangle map. Tarr, in Williams and others (1909), mapped and described glacial deposits and their origin in the adjacent Cayuga Inlet valley. Fairchild (1934) provided a comprehensive interpretation of deglacial events in western New York (with emphasis on the Cayuga Lake valley) including the sequence of regional proglacial lakes. Denny and Lyford (1963, plate 3) depicted Fairchild’s Valley Heads ice extent and deposits across the region. Mullins and others (1996) carried out seismic reflection surveys of Owasco Lake and seven other Finger Lakes and interpreted thickness of sediment fill and the deposits therein. Randall (2001) conceptualized and described the hydrogeologic framework of glacial aquifers within the local hydrophysiographic region as part of a larger report on the glaciated northeastern United States. Bird and Kozlowski (2014) and Bird (2014) used lidar elevation data to refine the extent of proglacial lakes described by Fairchild in the Cayuga County part of the study area. Bird and Kozlowski (2016a, 2016b) produced surficial geology maps of the Moravia and Sempronius 7.5-minute quadrangles. Most recently, Karig and Miller (2020) have documented features in the Cayuga Lake basin that imply northward drainage into the ice after the Brooktondale Readvance (a post Valley Heads readvance), which is counter to previous interpretations.
Data Sources and Methods
Surficial geology, subsurface deposits, and aquifer boundaries were based on well-log and test-boring data, the digital Soil-Survey Geographic database (SSURGO) (U.S. Department of Agriculture [USDA], 2008), lidar imagery, previously compiled geologic maps and reports, and field visits. The aquifer system boundary or aquifer extent is the extent of contiguous stratified-drift deposits within valleys, including mostly unsaturated deposits on adjacent hillside areas. Upland confined sand and gravel aquifers are limited in extent and potential productivity and are not included in this definition.
Well and test boring data used in this report are stored in the USGS National Water Information System (NWIS; USGS, 2022). A “CY” in the site name of the well or test boring indicates the well is located in Cayuga County, and a “TM” is for Tompkins County. Outside sources of well and test-boring records include the NYSDEC (which administers the New York State Water Well Drillers Registration Program), the New York State Department of Transportation, the Cayuga and Tompkins County health departments, and village or town records. All well and test boring data used in this report are compiled in Heisig (2023a).
The HVSR seismic soundings were completed at 31 sites to estimate the thickness of sediments overlying bedrock in the Owasco Inlet valley (Heisig, 2023b). The passive seismic method uses a single broadband three-component (x, y, and z directions) seismometer to record ambient seismic noise. The ratio of the averaged horizontal (x, y) to vertical (z) frequency spectrum is used to determine the fundamental site resonance frequency (f), which can be interpreted using a regression equation to estimate sediment thickness overlying bedrock (Lane and others, 2008).
At present (2021), two regression equations (Heisig and Fleisher, 2022) are used to estimate sediment thickness over bedrock. The first equation is for high shear-wave velocities in all thicknesses of sand and gravel or till, and mixed predominantly fine-grained stratified sediments greater than or equal to 275 ft thick:
and the second equation is for lower shear-wave velocities in mixed, predominantly fine-grained stratified sediments less than 275 ft thick: whereft
is sediment thickness over bedrock, in feet,
f
is fundamental site resonance frequency, in hertz (Hz),
R2
is the coefficient of determination.
Origin and Distribution of Glacial and Postglacial Deposits
The distribution of glacial and postglacial deposits is depicted on plate 1, the map in figure 2, and in seven hydrogeologic sections: A–A′ through G–G′ (location map, fig. 3; sections figs. 4–10, in back of report). Specifically, the thickness of the glacial deposits (overburden) atop bedrock in upland areas is shown in figure 2. Well and test-boring logs are provided in Heisig (2023a).
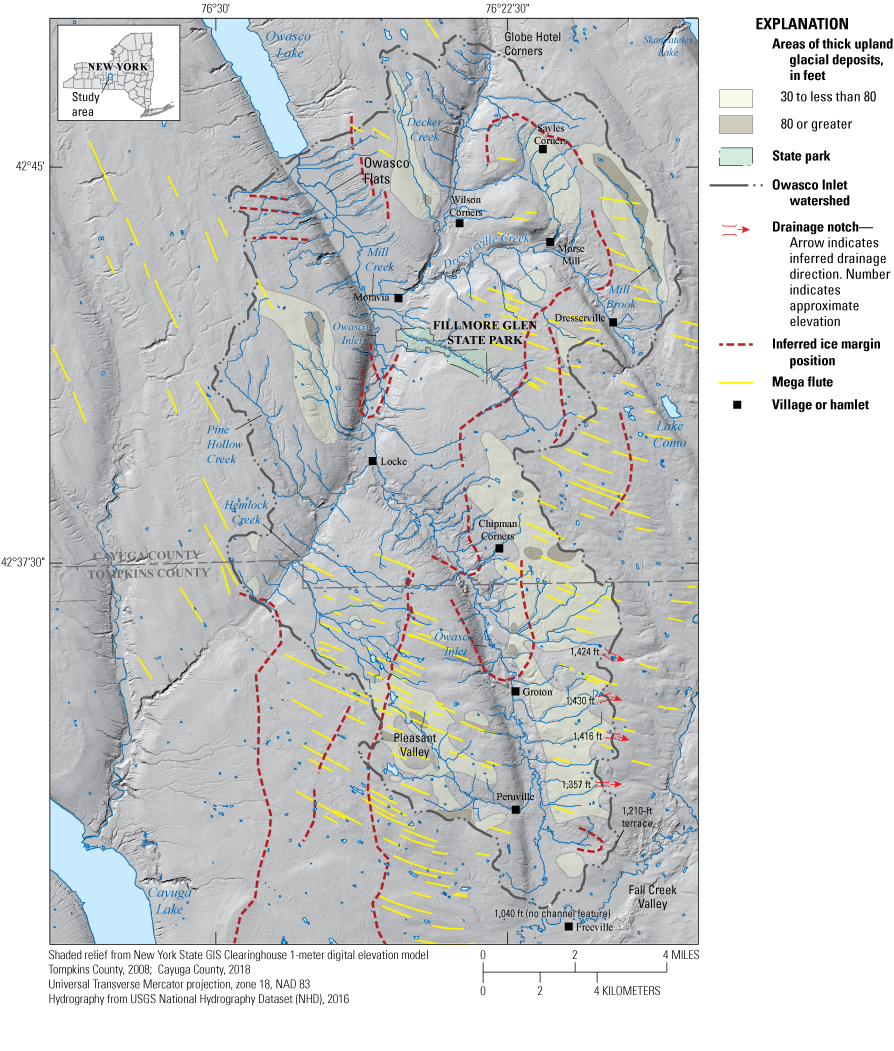
Map of the study area of the Owasco Inlet watershed, Cayuga and Tompkins Counties, New York, showing thick glacial deposits (mostly till) in upland areas, streamlined landforms (mega flutes) that indicate ice-flow directions, primarily from the north-northwest and west-northwest, and inferred ice positions.
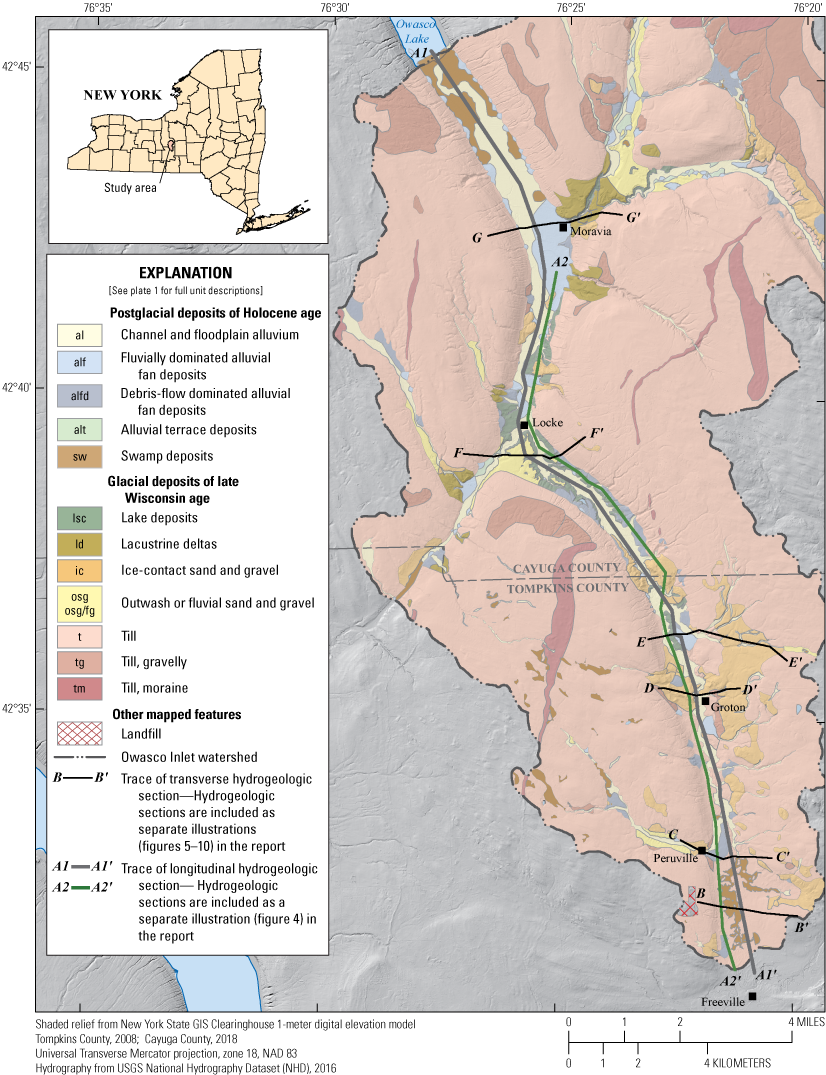
Location map of hydrogeologic sections, Owasco Inlet watershed, Cayuga and Tompkins Counties, New York.
Regional Overview
Most glacial deposits within the Finger Lakes region are derived from two primary Laurentide ice sheet advances and retreats during the late Wisconsin substage at the end of the Pleistocene Epoch. These include (1) the late Wisconsin last glacial maximum ice advance that extended as far south as Pennsylvania (Nissouri Stade, about 25,000 years before present [B.P.]) (Corbett and others, 2017; Stokes, 2017); (2) the Erie Interstade (retreat), peaking about 18,000 years B.P. (Dyke, 2004); and (3) the Port Bruce Stade, marked by the Valley Heads Readvance (Fairchild, 1932; detailed in Denny and Lyford, 1963, plate 3), which peaked about 17,000 calendar years ago, as extrapolated by Karig and Isacks (2019) from western New York (Muller and Calkin, 1993) and the Mohawk valley of east-central New York (Ridge, 2003). This readvance deposited moraines mostly in the form of outwash heads in valleys across much of western New York. The Owasco watershed was completely covered during both the last glacial maximum and the Valley Heads Readvance, and most surficial deposits were deposited during glacial advance to, and retreat from, the Valley Heads ice margin. Minor subsequent readvances, such as the Brooktondale (Karig, 2015), likely altered surficial geology in the southernmost part of the study area (Karig and Miller, 2020).
The Finger Lakes region is classified as part of the “northern rim of the Appalachian Plateau” hydrophysiographic region defined by Randall (2001), a region of high relief that sloped toward the ice sheet. This region has smooth uplands punctuated by deep Finger Lake valleys. Steep slopes upvalley (south) from deeply scoured Finger Lake troughs were an impediment to ice movement and to the free southward drainage of meltwater. Valley fill in this region is characterized by a predominance of thick fine-grained lacustrine deposits with or without intervening fine-grained till (or diamicton) units and a lack of surficial outwash deposits (Randall, 2001).
As the ice margin advanced or retreated through the Finger Lake troughs, the natural northward drainage was blocked, such that meltwater ponded in a series of proglacial lakes with initial outlets to the south, controlled by spillways at through-valley drainage divides at the heads of the Seneca and Cayuga valleys (Fairchild, 1934; Muller and Prest, 1985; Bird and Kozlowski, 2014; Bird, 2014). Karig (2015) and Karig and Miller (2020) have questioned that the ice margin acted as a dam after the Brooktondale Readvance (approximately [~] 16,300 calendar years ago) and suspect drainage into the ice, citing a lack of outlet channels at within-valley divides of some valleys and indications of northward drainage within the Six Mile Creek and Cayuga Inlet valleys.
It is generally accepted that lake levels subsequently dropped as northward ice retreat exposed isostatically depressed lower-elevation divides. As lake levels declined, the lower base levels caused stream incision and erosion of recently exposed glacial deposits with subsequent deposition in shallow residual lakes or exposed valley floors. With retreat of the ice margin, subsequent isostatic rebound has been greatest to the northeast (Lewis and others, 2005). Since the Finger Lakes drain northward, this greater isostatic rebound of the northern part of the Finger Lake basins has subsequently acted like a rising dam and increased the Finger Lake water levels, resulting in fine-grained postglacial deposition at the south end of the Finger Lake troughs (including the Owasco Lake trough) as described by Tarr (in Williams and others, 1909, p. 32) at Cayuga Lake.
Valley Heads Readvance and Retreat in the Owasco Watershed—Glacial and Postglacial Deposits
The ice that moved across the study area during the Valley Heads Readvance was part of the eastern edge of the Cayuga lobe (Fairchild, 1932; Denny and Lyford, 1963, plate 3; Karig and Miller, 2020, their fig. 2). The direction of ice movement, indicated by streamlined till (mega flutes; Clark, 1993) in upland areas (fig. 2), was initially parallel to southern Owasco Lake and the Owasco Inlet valley nearly as far south as Moravia, but shifted progressively more eastward until flow was almost due east at the southern edge of the study area (Freeville). Ice from the Cayuga lobe thus raked across much of the Owasco Inlet valley. A smaller number of less pronounced mega flutes continue eastward to the Fall Creek valley, smoothing over what appear to be ice-contact deposits. The Valley Heads (Fairchild, 1932) Readvance completely covered the Owasco Inlet watershed (fig. 2 of Karig and Miller, 2020) and extended several miles south and northwest of Freeville (fig. 2).
As Valley Heads ice thinned and the southeastern upland divide was exposed, a brief period of meltwater ponding occurred because of surrounding, stagnant ice, and eventual flow to the east likely took place across several notched divides (fig. 2): at 1,424-ft elevation (floored with coarse stratified sediments) east of Groton, an adjacent one immediately south at 1,430 ft, and less developed ones at 1,416 ft and 1,357 ft farther south, east and a little north of Peruville. As the ice continued to thin, it appears meltwater was conveyed southward along the east side of the watershed as indicated by scattered ice-contact deposits, ice-marginal drainage channels, and washed till (plate 1, figs. 7 and 8). The southeasternmost and lowest (~1,210 ft) drainage outlet with stratified material is the gap between the bedrock ridge of the southeastern divide and an isolated hill south of it (at the west end of section B–B′, on plate 1) at the junction with the broad Fall Creek valley, just northeast of Freeville (plate 1, fig. 2). It has the form of a small ice position (concave on ice side, complete with spring, transitioning to kame terrace or moraine that abuts extensive hummocky ice-contact deposits [a stagnant ice setting]) in the Fall Creek valley (plate 1). Carney (1909, p. 358) stated that the eastern side of the Owasco Inlet valley “is in general uniformly more gravelly” than on the west side and that the drift was amassed on that side by ice marginal drainage. Soil surveys and well logs support this observation (plate 1, figs. 7 and 8). Drainage likely continued northeast towards Cortland, N.Y. A similar asymmetric distribution of stratified deposits also occurs in the upper northwest-southeast trending Dresserville Creek valley (plate 1).
The lowest potential meltwater outlet at the Owasco Inlet headwater divide just north of Freeville is at ~1,040 ft elevation, but the surficial deposits are mostly till (underlain by lacustrine sediments) and there is no drainage channel. This indicates that ice persisted in the valley at this location (and perhaps as far as about 4 mi northward), as no lacustrine deposits have been observed over the till in soil borings and a test pit in the area (D. Karig, Cornell University, written commun., 2021).
Pauses in ice-margin retreat are best marked by moraines in the uplands and by accumulations of coarse stratified material (ice-contact deposits) in the valleys (plate 1, fig. 2). Both Carney (1909) and Bird and Kozlowski (2014) depict ice margins oriented southwest to northeast across the watershed, with small ice tongues in the main valleys. Ice margins in this and other valleys of the Finger Lakes basin impounded water in a series of proglacial lakes as proposed by Fairchild (1934) and subsequently refined by Muller and Prest (1985) and Bird and Kozlowski (2014). Thick fine-grained lacustrine deposits make up most of the valley fill in the Owasco Inlet valley (fig. 4). Sediment-laden meltwater discharge from beneath and within the ice into proglacial lakes resulted in the deposition of coarse-grained subaqueous fans at the ice margin that were eventually buried by finer lacustrine sand and then silt and clay as the ice margin retreated to the north (Randall, 2001). Deep sand and gravel beneath thick lacustrine fine sand, silt, and clay is common throughout the Owasco Inlet valley and to the south across the divide with Fall Creek at least into the village of Freeville (see all hydrogeologic sections [figs. 4–10], especially section A–A′ on plate 1, and fig. 4).
Very thick lacustrine deposits fill the inlet valley along two valley reaches between Locke and Groton (plate 1, figs. 8 and 9). From Locke southward about 2.5 mi, lacustrine deposits form dissected terraces as high as 65 ft above the floodplain and around 100 ft beneath it. A second localized area south of the one just described and 1 mi north of Groton has lacustrine deposits rising nearly 90 ft above the floodplain and capped by an alluvial fan.
Soils derived from lacustrine deposits are indicated by the USDA in the Owasco Inlet valley bottom between Freeville nearly to Groton (USDA, 2008). However, recent coring and a test pit in the valley bottom between Peruville and Freeville indicate a thin layer of ochre to brownish-gray till, derived from lacustrine deposits, overlying gray lacustrine deposits (fig. 11; Karig, 2022). Inspection of well logs in the area indicate surficial clay or hardpan between 6 and 50 ft thick and brown in color, when described, which is interpreted as till. This deposit represents a readvance (Brooktondale Readvance, Karig, 2015) during final retreat of ice from the area. The lack of lacustrine deposits above the till indicates that remnant ice likely occupied the valley or that there was no lake at least locally during final retreat.
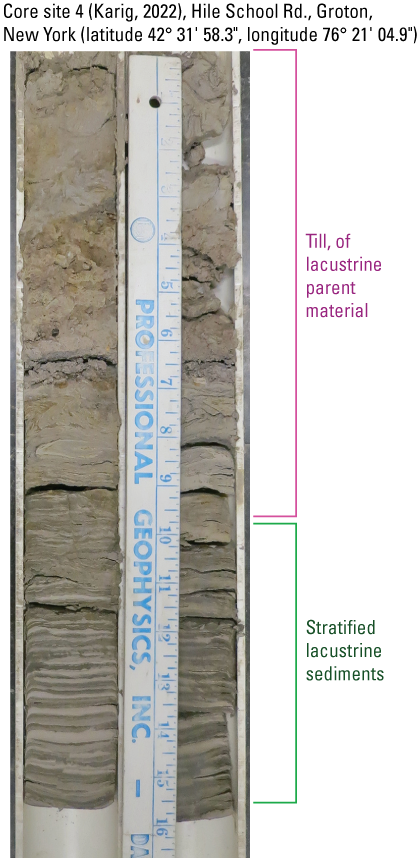
Photograph of Owasco Inlet valley piston core showing brownish till of lacustrine origin overlying stratified lacustrine sediments, Owasco Inlet watershed, Cayuga and Tompkins Counties, New York. Scale in inches. Photograph courtesy of Daniel Karig, Cornell University.
Pauses in retreat of the ice margin allowed buildup of coarse sediments from subaqueous or ice-marginal meltwater drainage into ice-contact deltas and development of lacustrine deltas from tributary streams (MacNish and Randall, 1982), which mark proglacial lake levels (Bird and Kozlowski, 2014). The most significant pause in ice retreat was at Groton, where the bedrock floor of the valley rises abruptly (fig. 4). Only at Groton are lacustrine deposits largely absent in the Owasco Inlet valley fill. Coarse stratified sediments make up most of the valley fill here, with the remainder either resedimented (flow) till or minor lacustrine deposits (figs. 4 and 7).
At other locations, it is uncertain that ice-contact deposits along valley walls are continuous from land surface downward through and beneath the lacustrine deposits, which may indicate ice readvance over lacustrine deposits. About 1 to 1.8 mi south of Moravia, just south of the transition to a narrower, shallower, and more southerly oriented valley reach (fig. 4), two features that rise as much as 40 and 75 ft, respectively, above the floodplain are identified as glaciofluvial by soil survey data (USDA, 2008) and Carney (1909). If these deposits predate the lacustrine deposits, a drape of lacustrine deposits (not evident) might be expected to overlie these deposits. The log from well CY 614 (plate 1, fig. 4) on the eastern feature indicates 20 ft of sandy clay underlain by 60 ft of lacustrine clay. This appears to indicate poorly sorted ice-contact sediments or till rather than glaciofluvial deposits at this location. The ice-contact delta about 3.1 mi south of Locke (Kozlowski and Graham, 2014; p. 31) likely spanned the valley and was later breached as lake levels declined. A test boring log (TM1083, plate 1, fig. 4) on the narrow floodplain between the east and west valley wall exposures of the ice-contact delta shows no subsurface indication of deltaic deposits—at least 105 ft of lacustrine fines (silty clay) beneath 6 ft of alluvium and shallow lake deposits (fig. 4). If the delta simply prograded over lacustrine fines, coarser (fine sand) lacustrine deposits might be expected below the delta at this location, as the delta was not far from the ice margin. Additionally, deformed lacustrine deposits exist near land surface beneath about 3 ft of coarse alluvial deposits at streambank exposures in Owasco Inlet about 1.3 mi south of Locke (fig. 12), which might indicate ice advance over the lacustrine deposits. Carney (1909) also described a similar outcrop and discussed possible evidence for glacial deposits older than the surficial deposits in the valley and surrounding area.
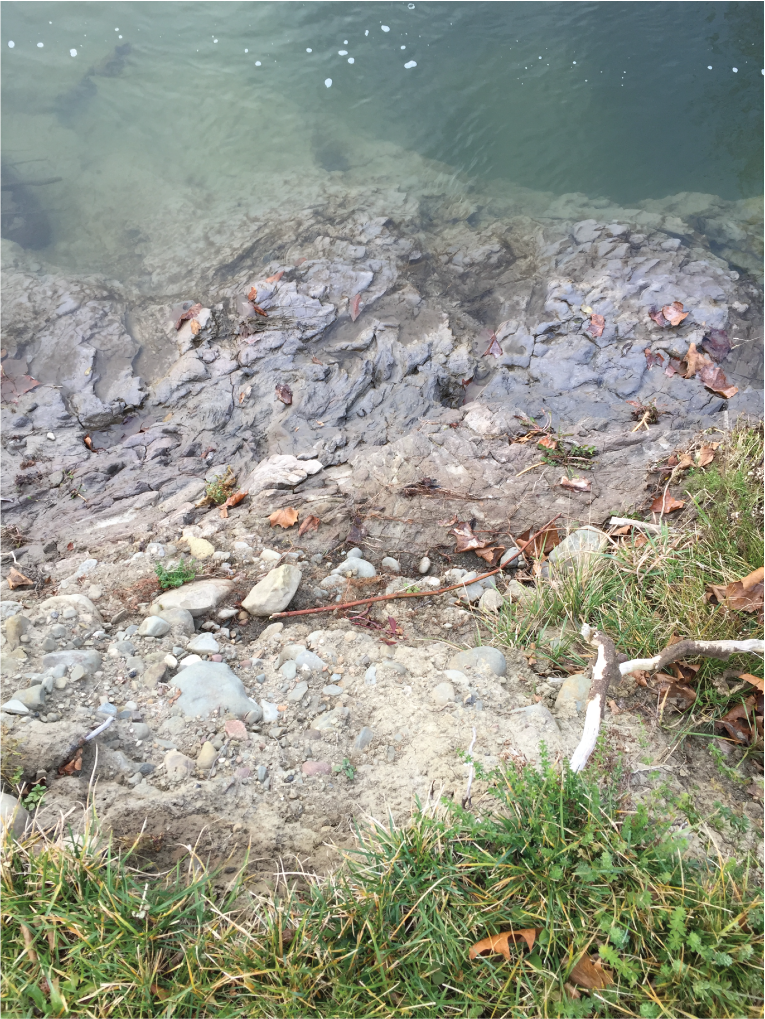
Photograph of deformed lake beds exposed on the east bank of Owasco Inlet south of Locke, New York. Photograph by Paul M. Heisig, U.S. Geological Survey.
Some delta-like features are outwash or fluvial gravel plains over fine-grained lacustrine deposits rather than complete deltaic sequences. The high terrace feature at the junction of the Owasco Inlet valley and the Hemlock Creek valley at Locke indicates inflow from the Hemlock Creek valley that deposited coarse fluvial or outwash sand and gravel over lacustrine fines (figs. 4 and 9). The feature is highest and coarsest near the Hemlock Creek valley. A homeowner indicated that his well (CY 149) is about 200 ft deep because a thick clay unit was penetrated beneath the surficial gravel. A gravel pit along the northwest edge of the feature penetrated clay beneath very coarse gravel that facilitated creation of a pond. Likewise, another pond and contact springs are evident on the east side of this feature.
Another gravel plain over lacustrine fines is at the junction of the Dresserville Creek and Decker Creek valleys east of Moravia. Most of the coarse-grained sediment was derived from southward drainage from ice margin retreating up, and at the head of, Decker Creek valley. This gravel plain merges into thick deltaic deposits at the confluence of the Decker Creek and Dresserville Creek valleys with the Owasco Inlet valley at Moravia. About 15 ft of gravel overlays fines in a played-out gravel pit at the northernmost edge of where the tributary valleys join (south of Wilson Corners), and 0.2 mi due west, a well log (CY1604, plate 1) indicates 20 ft of sand and gravel over 40 ft of gray clay.
Ice in the Owasco Inlet watershed was part of the eastern flank of the Cayuga ice lobe centered in the Cayuga trough (Fairchild, 1932; see Denny and Lyford, 1963, plate 3; Karig and Miller, 2020). Thus, ice retreat across the watershed was toward the Cayuga trough—nearly due west in the south, and more northwestward to the north as indicated by traces of moraines (fig. 2, plate 1; Carney, 1909; Bird and Kozlowski, 2014). This orientation is best illustrated by the upland moraines that can be traced to the small valley tongue associated with the ice-contact delta about 3.7 mi south of Locke (fig. 2). As ice retreated northward in the valley reach from Freeville to Locke, meltwater drained over an unvegetated and unstable landscape toward the valley until the margin retreated west of the watershed divide. Sediments conveyed by the largest drainage pathway from the ice margin (Pleasant Valley, plate 1, fig. 2) created a large fan feature at Peruville. Carney (1909, p. 341) stated that the large alluvial fan and small upstream gorge at Peruville are out of proportion with the small drainage area of the present-day stream and thus represent abnormal drainage conditions—most likely drainage from when the ice was “near at hand.” This feature has a more irregular surface than large, present-day alluvial fans such as the two at Moravia (plate 1) and is incised by the current stream, which indicates they are older features. Church and Ryder (1972) describe fans deposited during the early part of the paraglacial (recently deglaciated) period as more debris-flow rich than fluvial. Therefore, the term “debris-flow dominated alluvial fan” (alfd map unit) is used for these deposits in this report. These features are noted in smaller upland drainages such as Pine Hollow Creek, Pleasant Valley (upgradient of Peruville), and the drainage south of Sayles Corners (plate 1, fig. 2).
Apart from the large fan at Peruville, small fan features exist at the base of steep drainage channels on the walls of the Owasco Inlet valley. Where drainages are closely spaced, the smaller features form an apron along the base of the valley wall. The alfd units are not well defined and may include adjacent till or slumped till, likely derived from lacustrine deposits. These units and adjoining deposits mapped as till have variable soil survey parent materials (glaciofluvial, till, or lacustrine), and well logs report clay, hardpan with gravel layers, and clayey, dirty, or clean gravel. There are no detailed geologic logs of these materials. Without such geologic control, deposits at the base of the valley wall areas mapped as lacustrine silt and clay, till or alfd are speculative, especially as till is likely derived from mostly lacustrine deposits.
Retreat of the ice and exposure of lower lake outlets (Bird, 2014; Bird and Kozlowski, 2014) led to periodic drops in proglacial lake levels and incision of Owasco Inlet valley, especially between Locke and Groton, where lacustrine deposits and the ice-contact delta are 60–90 ft to nearly 200 ft, respectively, above the present-day floodplain. Lowering of base level led to incision in the other drainages within the watershed and deposition of numerous small alluvial fans in the valleys. Two large alluvial fans from Mill Creek (the Decker and Dresserville Creek watersheds) and from the stream at Fillmore Glen State Park have merged and dominate the valley bottom at Moravia. From Moravia north to Owasco Lake, Owasco Flats is a mix of floodplain and swamp lands, with at least 25 ft of organic, fine-grained alluvial deposition over a basal coarser grained deposit that is early alluvium or of alluvial fan origin, as noted at Moravia in figure 4.
At the southern end of the watershed, near the divide north of Freeville, the valley floor is also largely swamp, much of which is underlain by a thin layer of till, over lacustrine deposits. This valley area has probably changed least in postglacial time because of low relief and high elevation (limited drainage area).
Groundwater Resources
Groundwater is the sole source for public and domestic water supply within the Owasco Inlet watershed. Sand and gravel aquifers are most commonly tapped in the Owasco Inlet valley and in some of the larger tributary valleys, whereas fractured bedrock aquifers are most commonly used in upland areas. Current (2016) municipal supplies serving the villages of Moravia, Locke, and Groton all tap sand and gravel aquifers.
Sand and Gravel Aquifer Type and Distribution
Several sand and gravel aquifer settings are recognized within the Owasco watershed: confined (including confined by till), semiconfined, and unconfined/semiconfined aquifers within the Owasco Inlet valley, and unconfined and confined aquifers in upland areas (fig. 13). The distributions of these aquifers (especially confined and semiconfined) within delineated areas are not continuous—they may be locally absent or poorly sorted (of poor permeability).
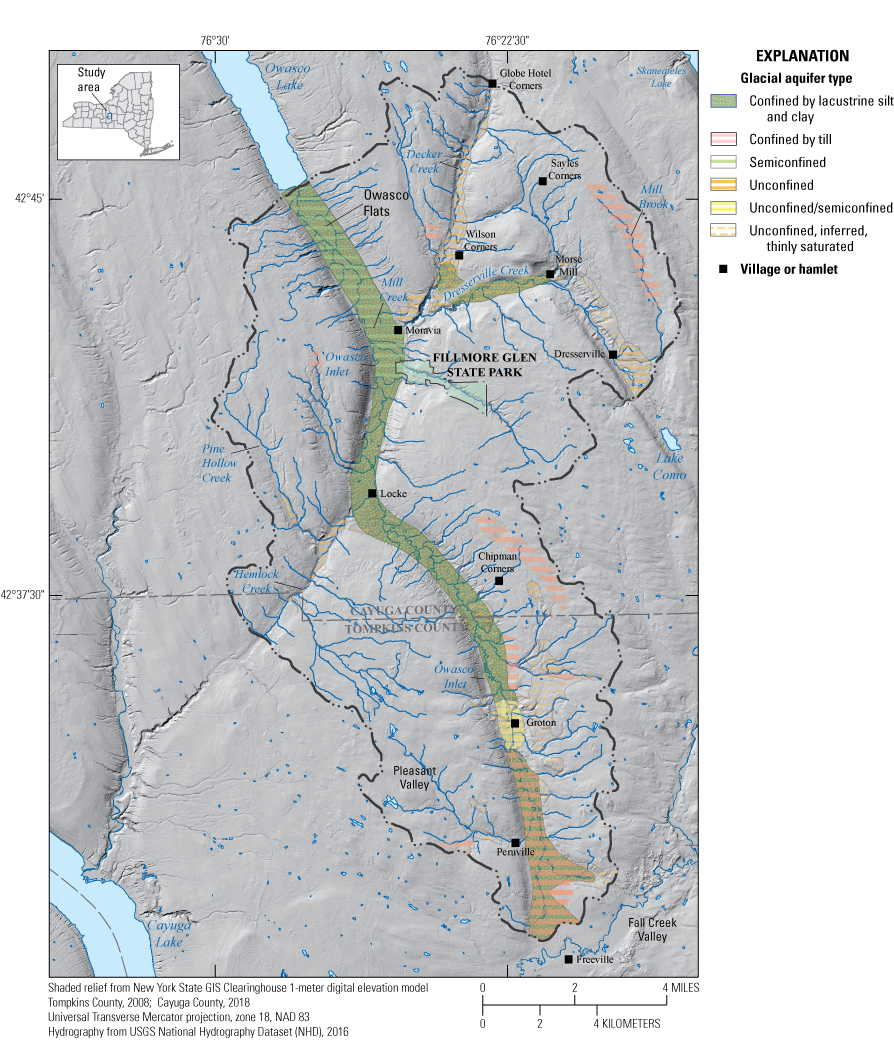
Map of Owasco Inlet watershed study area showing distribution of glacial aquifer types, Cayuga and Thompkins Counties, New York.
Unconfined aquifers are the most susceptible to contamination from nearby activities at land surface because precipitation and subsequent recharge can transport contaminants directly through the unsaturated zone to the water table. Confined aquifers are less susceptible to contamination from local land surface because confining units largely prevent downward movement of water. Recharge of confined aquifers occurs elsewhere—at unconfined upvalley locations and along valley walls where alluvial fans, ice-contact deposits, or stream incision into the valley wall may provide pathways for downward movement of groundwater.
A deep, confined aquifer underlies much of the Owasco Inlet valley within the study area (figs. 4 and 13). A smaller, separate, and largely untested confined aquifer is also present in the lower reach of Dresserville Creek. The Owasco Inlet valley confined aquifer is known to extend south and likely north of the study area. Aquifer material extends south across the low divide with Fall Creek at least to Freeville, where flowing artesian conditions exist in many domestic wells. The aquifer also likely continues north of Moravia and beneath Owasco Lake, as northward ice retreat continued to generate sediment-laden meltwater that was deposited as subaqueous fans immediately in front of the ice or as eskers within the ice, which may have fed the subaqueous fans. Water-borne seismic surveys of the Finger Lakes conducted by Mullins and others (1996) lend support to this interpretation. Owasco Lake and other Finger Lakes commonly showed chaotic reflectors that form hummock and ridge features above the bedrock and below lacustrine deposits at the north ends of lakes that were interpreted as eskers or ice-contact deposits. These units transitioned into more regular reflections to the south that were interpreted as more sorted, finer grained “subaqueous outwash” units.
The thickness of this aquifer is rarely documented because drillers obtain sufficient water for domestic purposes shortly after reaching it and thus do not drill to bedrock. Aquifer thicknesses are generally estimated to be 20 ft or less from HVSR depth-to-bedrock estimates and well logs in the Owasco Inlet valley (fig. 4).
Hydraulic heads in the aquifer have historically been flowing artesian—above floodplain elevation in the valley bottom—especially north of Groton (the Locke area in particular) and at the south-end drainage divide and beyond in the Freeville area. Some estimates of head from New York State Department of Transportation borings in the Owasco Inlet valley since the 1950s were 100 ft above land surface with flow rates as high as 150 gallons per minute. These conditions indicate that drillers should expect and be prepared to control high water pressures in this aquifer.
The Village of Locke’s water supply taps this aquifer and serves a population of 600 (U.S. Environmental Protection Agency [EPA], 2017). The two production wells are 179 and 194 ft deep. Total water withdrawn through these wells in 2015 was about 10.7 million gallons (29,300 gallons per day [gal/d]; NYSDEC, 2016). The yields of these wells were not reported.
The Semiconfined Aquifer Above the Lacustrine Deposits
A shallow, semiconfined aquifer exists above the lacustrine deposits and beneath recent fine-grained alluvial or lacustrine deposits within about the lower (northernmost) 5 mi of the Owasco Inlet valley (figs. 4 and 13). This aquifer was likely deposited by northward drainage during a low stand of Owasco Lake along with early alluvial fan deposition from the Mill Creek drainage (below the confluence of Decker and Dresserville Creeks). Tributary stream water infiltration into alluvial fans (Randall, 1978) is likely a significant source of recharge to the aquifer. Infiltration from the Owasco Inlet into the aquifer is another possible water source, especially where the Owasco Inlet has impinged against the valley wall, where alluvial fan or other permeable deposits or fractured bedrock may provide an avenue for induced downward groundwater flow.
The Village of Moravia’s water supply taps this aquifer and serves a population of 1,950 (EPA, 2017). It is a thin (~15 ft) but productive aquifer about 20 to 35 ft below land surface, overlain by fine alluvium and underlain by thick, fine lacustrine deposits. For example, see test well CY 109 on plate 1. Production wells each yield about 400–500 gal/min. Total water withdrawn from the aquifer in 2015 was about 161 million gallons (440,000 gal/d; NYSDEC, 2016).
Unconfined or Unconfined/Semiconfined Aquifers Within the Owasco Inlet, Decker Creek and Hemlock Creek Valleys
Four unconfined or unconfined/semiconfined valley aquifers exist within the study area: (1) a short segment of Owasco Inlet valley at the Village of Groton, (2) the upper 70 or 80 percent of the Decker Creek valley, (3) much of the lower Hemlock Creek valley, and (4) a short segment of Dresserville Creek valley at Dresserville (fig. 13). Ice retreat paused at Groton long enough for a buildup of ice-contact sand and gravel with some intervening poorly sorted intervals in the valley (up to about 120 ft thick) and up the adjacent valley walls (fig. 7). This is the only place in the Owasco Inlet valley where lacustrine deposits are largely absent. The Decker Creek valley conveyed meltwater discharge from ice at its drainage divide. This discharge resulted in deposition of up to 90 ft of sand and gravel fill within this valley and deposition of the extensive deltaic deposits at Moravia (fig. 10, plate 1). Hemlock Creek valley (divide elevation about 957 ft) connected the Owasco Inlet basin with the Cayuga Lake basin during early proglacial lake phases (Fairchild, 1934; Bird and Kozlowski, 2014). Only a thin deposit of fluvial gravel is present above bedrock in the valley bottom or atop lacustrine deposits at the junction with the Owasco Inlet valley (fig. 9). The thickness of saturated ice-contact sand and gravel in the valley at Dresserville is unknown (fig. 13). These aquifers are likely in hydraulic connection with adjacent confined aquifers within the Owasco Inlet and Decker Creek valleys and thus might become sources of recharge if the deeper aquifers are used for groundwater withdrawals. Furthermore, where streams cross unconfined aquifers, pumping wells may induce infiltration of stream water into the aquifer.
A few unconfined sand and gravel deposits of small extent in, or immediately adjacent to, valleys are also depicted in figure 13. They are likely thinly saturated, and thus limited as primary sources of groundwater, although they can contribute recharge to deeper valley deposits if hydraulically connected.
The Village of Groton draws part of its water supply from two production wells (120 and 125 ft deep) that tap the unconfined aquifer in the Owasco Inlet valley. Both production wells have pumps rated at 250 gal/min (C. Shurtleff, Department of Public Works Supervisor, Village of Groton, oral commun., July 5, 2017). The absence of thick lacustrine deposits indicates semiconfined or unconfined conditions in the valley fill. Additionally, the presence of sand and gravel extending up the valley walls at Groton allows for greater potential aquifer recharge than elsewhere in the valley (figs. 4 and 7). The favorability for recharge also means that the aquifer is more susceptible to contamination from human activities at land surface. Leachate from past and current (2016) storage of road-deicing salts in a former gravel pit on the west side of the valley above the village (plate 1, fig. 7) likely affects water quality at the wellfield. Specific conductance of a water sample from a production well (TM 931; site ID 423523076220902) in 2017 was 922 microsiemens per centimeter at 25 degrees Celsius (USGS, 2022), which is about double the specific conductance of a confined-aquifer water sample collected near Locke. Sodium and chloride were also elevated (83.9 milligrams per liter [mg/L] and 162 mg/L, respectively). The sodium concentration was above the 20 mg/L health guideline for people on sodium-restricted diets (U.S. Environmental Protection Agency, 2012), but the water is blended with another water source to reduce the concentration in tap water.
The Decker Creek valley unconfined aquifer is currently only used for domestic supplies. Well logs indicate about 60 ft of saturated sand and gravel at the south end of the aquifer. This area has the greatest unconfined aquifer water-resource potential outside of the Groton area. The Decker Creek valley aquifer has less potential northward toward the divide as the valley narrows. Valley sediment-fill thickness likely thins northward, but there are no well-log data in this area to confirm this.
The Hemlock Creek valley aquifer has the lowest water-resource potential as fluvial gravels are likely unsaturated and sediment fill in the valley bottom is thin where known.
Unconfined Upland Aquifers
Upland unconfined aquifers mostly represent deposition by ice-marginal drainage. Thicknesses range from a veneer of gravel to at least 60 ft. These deposits are typically limited in area and may or may not support domestic wells. However, a municipal supply utilizes this resource with a large infiltration gallery.
The Village of Groton’s primary source of water is the Morton Works, an upland infiltration gallery, in operation since the 1890s, which draws water from a thin sand and gravel outwash deposit near a notched divide with the Fall Creek drainage. The water is gravity-fed to the village. This source, together with the two wells in the valley, serves a population of 2,470 (EPA, 2017). Total water produced by both supplies in 2015 was about 130 million gallons (356,000 gal/d) (NYSDEC, 2016). The Morton Works is the primary source, with a flow of about 220 gal/min (Tompkins County Department of Health, unpub. data, 2017) that may exceed 300 gal/min after periods of significant rainfall (C. Shurtleff, Department of Public Works Supervisor, Village of Groton, unpub. data, 2017). Protection of watersheds of such shallow, unconfined sources is particularly important for maintaining water quality.
Confined Upland Aquifers (Till Confining Unit)
Upland sand and gravel aquifers are overlain by till between 20 and 136 ft thick in seven locations within the study area (fig. 13). This implies at least local ice readvance over stratified deposits. The four of largest areal extent are on the east side of the watershed, where the upland glacial deposits are generally thickest (fig. 2). The two largest areas are along the eastern edge of the watershed and parallel the northwest-to-southwest alignment of Owasco Inlet valley north of Moravia and the Dresserville Creek valley southeast of Morse Mill (fig. 13). Two wells tapping confined sand and gravel in the northern area, along the Mill Brook valley (hamlet of Sempronius) did not reach bedrock after 162 and 91 ft; a third well reached bedrock at 98 ft (wells CY 473, CY 518, and CY1324, respectively). All wells are completed in 13 to 26 ft of gravel. The southern area is near the crest of the divide with the Fall Creek watershed, northeast of Chipman Corners (fig. 12). Two wells in this area (CY 477, CY1409) were completed in sand and gravel at 20 and 38 ft beneath till, respectively, although a third well (CY1186) was completed at 115 ft and recorded 95 ft of stratified material beneath about 20 ft of till (plate 1). Well CY1186 may hint at a west-to-east buried notch to the Fall Creek watershed.
Reported domestic well yields from these areas are between 6 and 20 gal/min. The water-resource potential for anything larger than domestic supply is unlikely, depending on the degree of confinement. If strongly confined, little recharge may replenish groundwater withdrawals and thus limit well yields.
Groundwater Recharge, Flow, and Vulnerability to Contamination
Groundwater recharge, flow in the subsurface, and discharge to low points on land surface is the subsurface part of the hydrologic cycle. Groundwater recharge is rainfall and snowmelt that infiltrates land surface, moves through the unsaturated zone, and joins groundwater at the water table—the top of the zone of saturated sediments or fractured bedrock. During a given year, the addition of water to the groundwater system induces groundwater to flow in directions of lower hydraulic head, and to ultimately discharge to low points on land surface that act as drains (streams, lakes, wetlands, and springs; fig. 14). If groundwater did not flow and discharge, water levels would rise and eventually flood land surface. Groundwater flows through aquifers, which are water-saturated sediments or rock that are thick and permeable enough to supply water to wells, and through materials that are too thin or low in permeability to be considered aquifers. Groundwater residence times can be relatively short (months, years), such as recharge from precipitation on valley bottoms that flows within floodplain sediments a short distance and discharges to the valley stream. Other groundwater residence times may be very long (hundreds to thousands of years) in some regional aquifers (Walter and others, 2020) and possibly in local, highly confined aquifers.
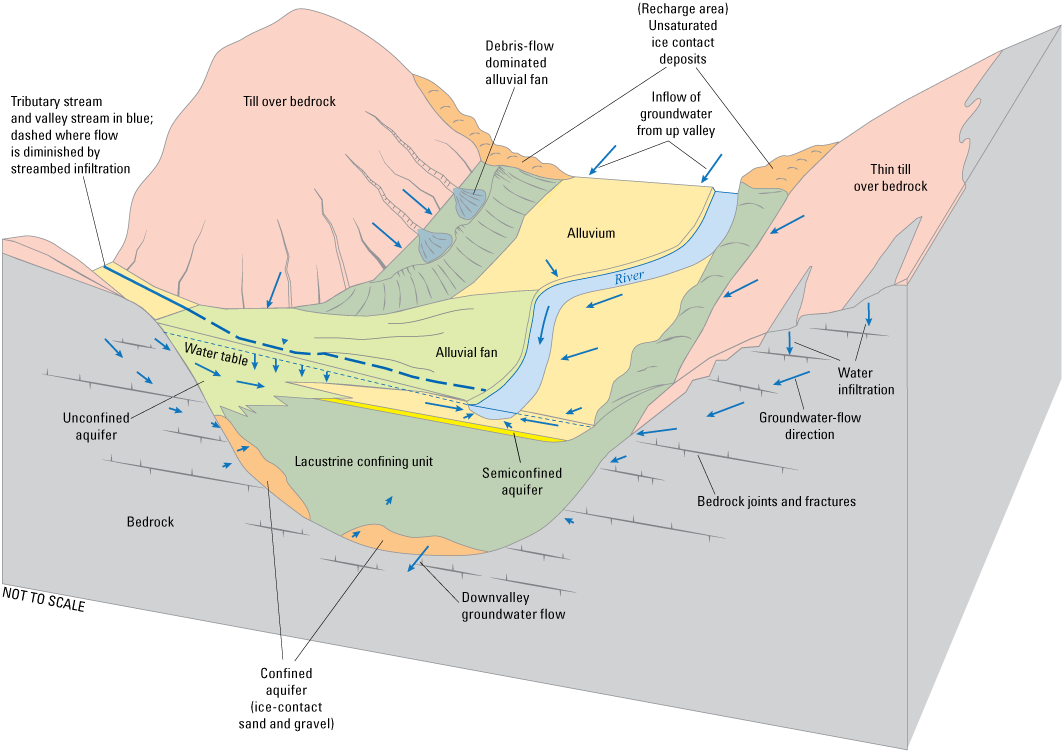
Conceptual block diagram of groundwater flow and aquifers in the Owasco Inlet valley, Cayuga and Tompkins Counties, New York.
Recharge can only occur where the sediments or bedrock at and below land surface are permeable enough to allow infiltration. In the Owasco Inlet watershed, for example, thick glacial till and fine-grained lacustrine deposits have low permeability and thus allow little, if any, recharge, whereas stratified coarse-grained deposits (ice-contact, outwash, and some coarser alluvium and alluvial fans) can be areas of high recharge (fig. 14). Fractured bedrock can vary widely in permeability, but in general it is less permeable than coarse stratified sediments; most fracture permeability in clastic (noncarbonate) sedimentary rock in hillside areas is approximately within the upper 100 ft of bedrock (fig. 14; see Heisig, 1999, p. 46 for an example). Recharge is most likely to occur in upland areas with thin, discontinuous till over bedrock, but recharge in upland areas of thick till is likely close to nil. Thus, upland sand and gravel aquifers confined by thick till are probably a limited resource.
Unconfined and semiconfined sand and gravel aquifers in valleys may receive recharge from direct precipitation and two additional sources: infiltration of streamwater through alluvial fan deposits where tributary streams enter valleys (Randall, 1978), and unchanneled surface runoff or subsurface groundwater flow from adjacent, unchanneled valley walls into valley-fill deposits (Morrissey and others, 1988). A fourth, and potentially major, source of recharge to these aquifers is induced infiltration of stream or river water into aquifers where groundwater withdrawals from wellfields intercept, and then reverse, natural groundwater flow to the stream. This may account for the high well yields at the Moravia wellfield.
Confined aquifers receive recharge indirectly through hydraulic connection with permeable deposits (sand and gravel, or bedrock), either in valley reaches where stratified sand and gravel are the primary valley fill (unconfined aquifers) such as at Groton, or where ice-contact, deltaic, or alluvial fan deposits along valley walls extend down to the confined aquifer (figs. 7 and 14).
The likelihood of groundwater contamination from human activities at land surface is highest in unconfined aquifers since infiltrating water (including induced infiltration) can move through the unsaturated zone directly to the aquifer. Upland areas of thin till may also provide short pathways for contaminants into the underlying fractured bedrock. Common contaminants include road-deicing salts, spills along roadways, and septic leachate. Landfills may also be a source of leachate to groundwater if protective caps or liners fail. Supply wells in unconfined and semiconfined aquifers that induce water from rivers are susceptible to negative changes in river water quality such as turbidity, algal blooms, and uncontrolled wastewater discharges.
Confined aquifers are more protected from contamination by overlying land-use activities because the confining unit prevents downward groundwater flow. For example, contaminants infiltrating from land surface in a valley floodplain underlain by fine lacustrine deposits (fig. 14) will be entrained by shallow groundwater flow in the alluvium and eventually discharge to the valley stream. Confined aquifers are susceptible to contamination from activities in their recharge areas—preventing high-contamination-risk land uses in the most likely recharge areas can help safeguard these aquifers.
Summary
Glacial deposits and distribution and type of sand and gravel aquifers were delineated within the 117-square-mile Owasco Inlet watershed in Cayuga and Tompkins Counties, New York, as part of a long-term detailed aquifer mapping program between the U.S. Geological Survey and the New York State Department of Environmental Conservation. Well and test-boring logs were compiled from various sources and horizontal-to-vertical spectral ratio seismic soundings were carried out to estimate bedrock depths in the Owasco Inlet valley. These data, along with existing soil and surficial material mapping, light detection and ranging (lidar) imagery, and a lidar digital elevation model were used to prepare seven hydrogeologic sections and a hydrogeology map plate.
The Owasco Inlet watershed is similar in form to other watersheds of the Finger Lakes region of New York State, characterized by broad, smooth uplands punctuated by the 18-miles-long Owasco Inlet valley and the secondary valleys of Decker, Dresserville, and Hemlock Creek. All these streams occupy “through valleys,” whose former upland divides have been completely removed—the valleys are continuous into adjacent watersheds such that watershed divides are high points within the valley bottoms.
Most surficial deposits within the Owasco watershed are derived from the advance and subsequent retreat from the Valley Heads ice margin. Ice of the Cayuga lobe covered the watershed, with ice flowing into the northern part of the watershed from the northwest, parallel to Owasco Lake, but with ice in the southern two-thirds progressively flowing more from the west to the east. The Owasco Inlet watershed drains north, like other Finger Lakes watersheds, and during retreat from the Valley Heads ice margin, northward drainage was largely blocked by the ice, which resulted in development of a series of regional proglacial lakes and widespread deposition of fine lacustrine sediments in the Owasco Inlet valley. These sediments are commonly underlain by thinner coarse-grained stratified deposits (subaqueous fans and eskers) of variable sorting and permeability. The only place where fine-grained deposits are largely absent in the Owasco Inlet valley is at Groton, N.Y., where the ice paused long enough for coarse-grained sediments to fill much of the valley. South of Groton, and especially south of Peruville, a relatively thin layer of till (derived from lacustrine deposits) acts as an additional confining layer over lacustrine deposits. Smaller, higher elevation valleys have a wider variety of glacial valley-fill deposits, ranging from sand and gravel to till.
Groundwater is the sole source of water supply in the Owasco Inlet watershed. Sand and gravel aquifers are most commonly tapped in the Owasco Inlet and Decker Creek valleys, and fractured bedrock is most commonly tapped in the uplands and the other valleys. All municipal supplies draw from glacial sand and gravel aquifers that are within or adjacent to the Owasco Inlet valley. Confined, semiconfined and unconfined aquifers exist within in the valley fill. Upland unconfined and confined (by till) sand and gravel aquifers were identified from domestic well logs, but they are limited in extent and likely limited in the amount of recharge they receive.
The deep confined aquifer, beneath the fine lacustrine sediments, stretches the entire length of the Owasco Inlet valley. The Town of Locke is the only municipality that taps this aquifer for water supply.
A thin semiconfined aquifer at Moravia presumably extends north to Owasco Lake and a short distance south. This shallow aquifer overlies proglacial lake deposits and is partly confined by overlying recent lake deposits and alluvium, and alluvial fan deposits. Withdrawals from this aquifer may also induce water from the Owasco Inlet into the aquifer, as the wells tapping this aquifer are the most productive within the watershed. The Village of Moravia draws from this aquifer.
The Village of Groton draws from two aquifers: (1) a thin upland unconfined sand and gravel aquifer tapped with an infiltration gallery and (2) the unconfined or semiconfined section of the Owasco Inlet valley at Groton.
Another aquifer with potential for municipal supply is the unconfined aquifer in the Decker Creek valley near Wilson Corners. Confined aquifers in the lower and uppermost Dresserville Creek valley and an unconfined aquifer near Dresserville may have some water-resource potential, but they are largely untested.
Unconfined aquifers are the most susceptible to contamination from activities at land surface because precipitation and subsequent recharge can transport contaminants directly to the water table. Confined aquifers are less susceptible to contamination from overlying land surface because confining units largely prevent downward movement of water. Recharge occurs elsewhere—at unconfined upvalley locations and along valley walls where alluvial fans, ice-contact deposits, or valley stream incision into the valley wall may provide pathways for downward movement of groundwater.
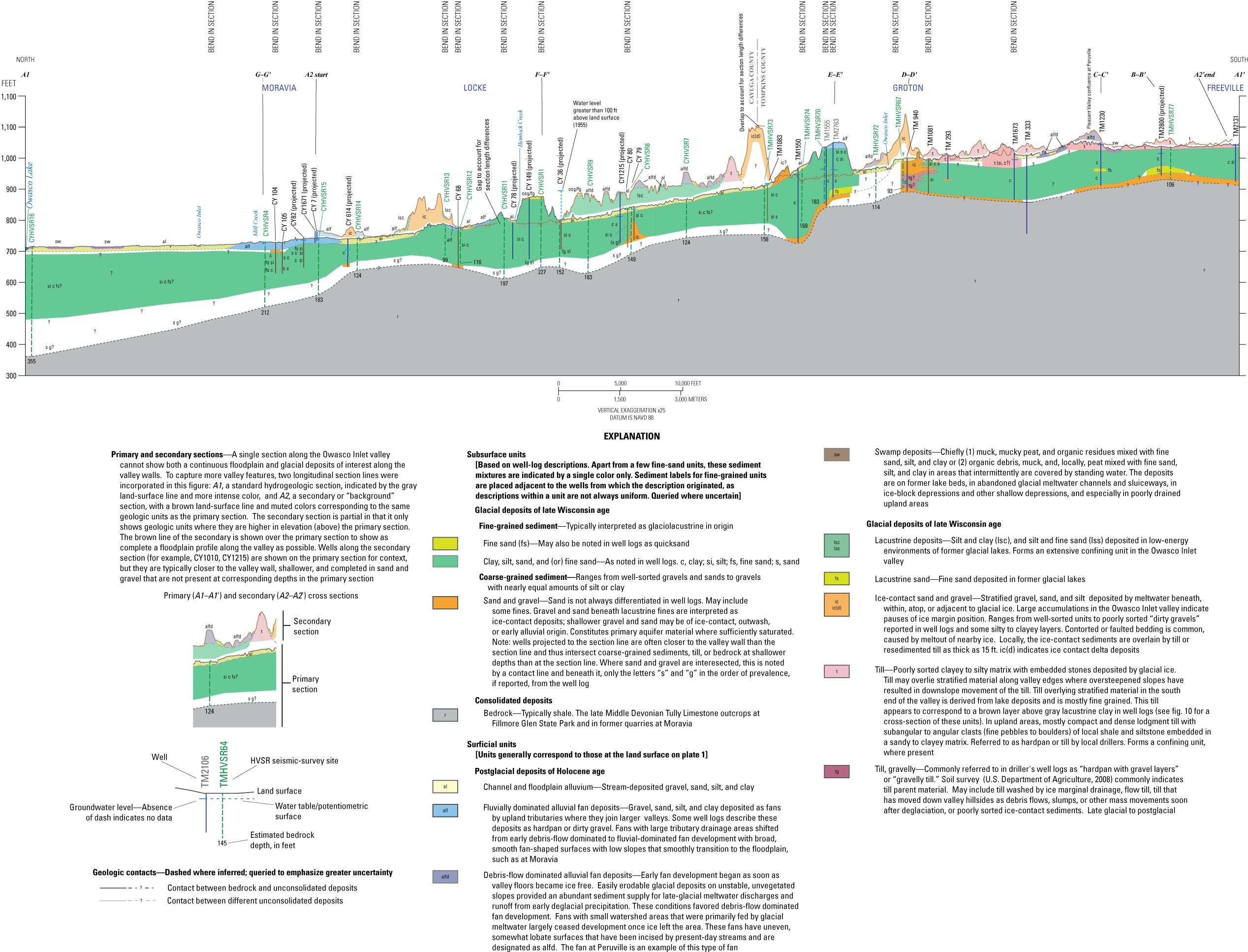
Longitudinal hydrogeologic sections A1–A1′ and A2–A2′, spanning the length of the Owasco Inlet valley, Tompkins and Cayuga Counties, New York. Figure 4 is available as an oversize file at https://doi.org/10.3133/sir20235031. For location of cross section, wells, and other labeled features, see plate 1. HVSR, horizontal-to-vertical spectral ratio; NAVD, North American Vertical Datum of 1988.
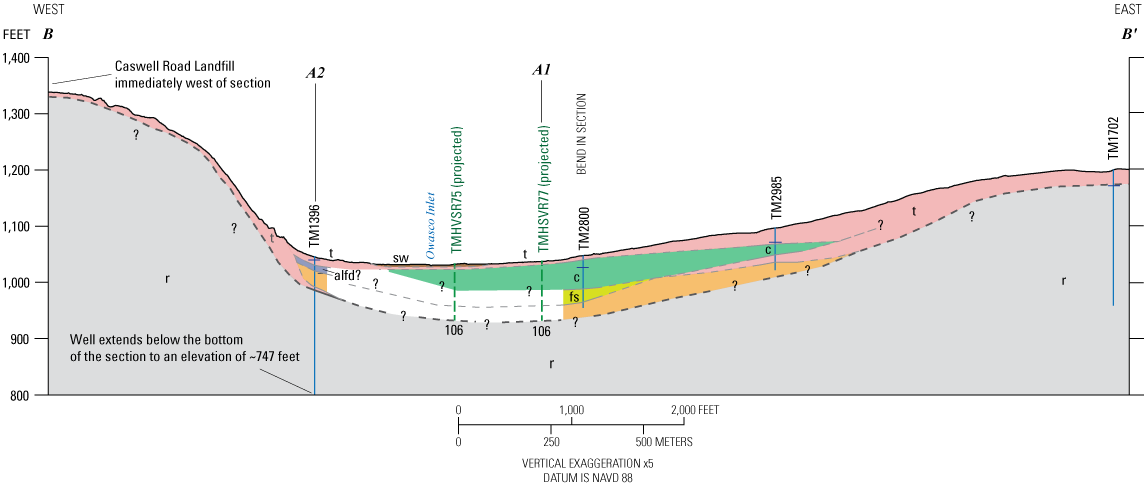
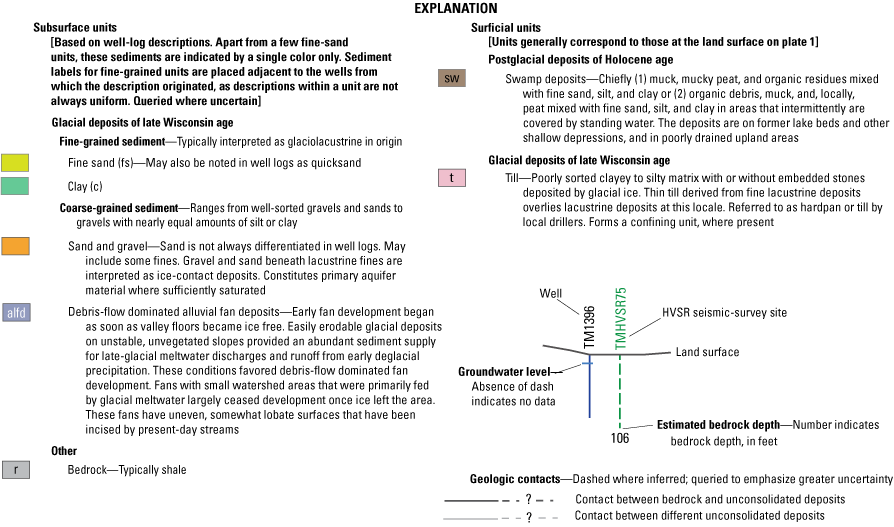
Hydrogeologic section B–B′ across the Owasco Inlet valley near Hile School Road, between Freeville and Peruville, Tompkins County, New York. For location of cross section, wells, and other labeled features, see plate 1. HVSR, horizontal-to-vertical spectral ratio; NAVD, North American Vertical Datum of 1988.
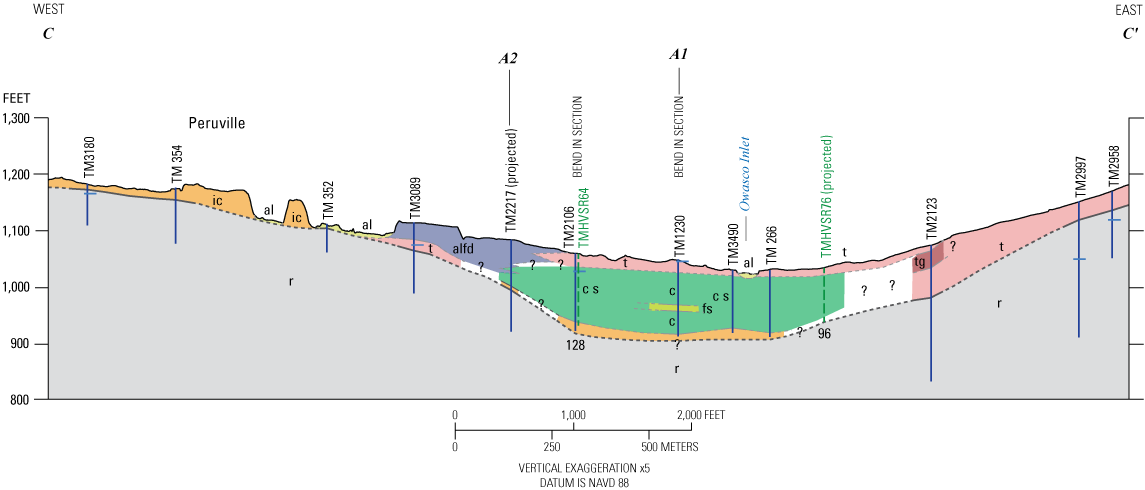

Hydrogeologic section C–C′ across the Owasco Inlet valley at Peruville, Tompkins County, New York. For location of cross section, wells, and other labeled features, see plate 1. HVSR, horizontal-to-vertical spectral ratio; NAVD, North American Vertical Datum of 1988; ND, no data; ~, approximately.
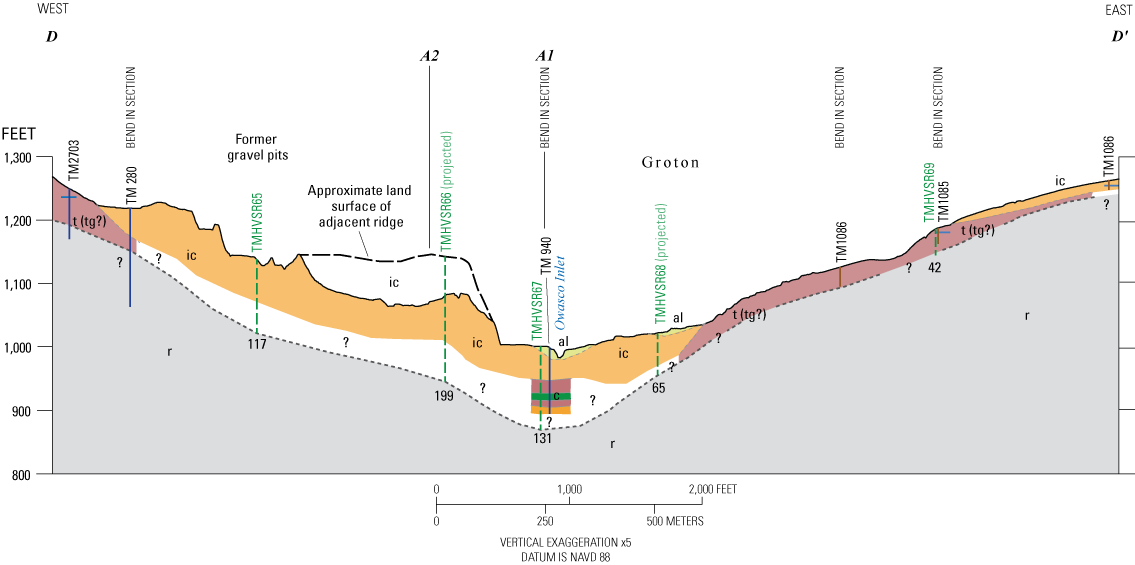

Hydrogeologic section D–D′ across the Owasco Inlet valley at Groton, Tompkins County, New York. For location of cross section, wells, and other labeled features, see plate 1. HVSR, horizontal-to-vertical spectral ratio; NAVD, North American Vertical Datum of 1988; ND, no data.
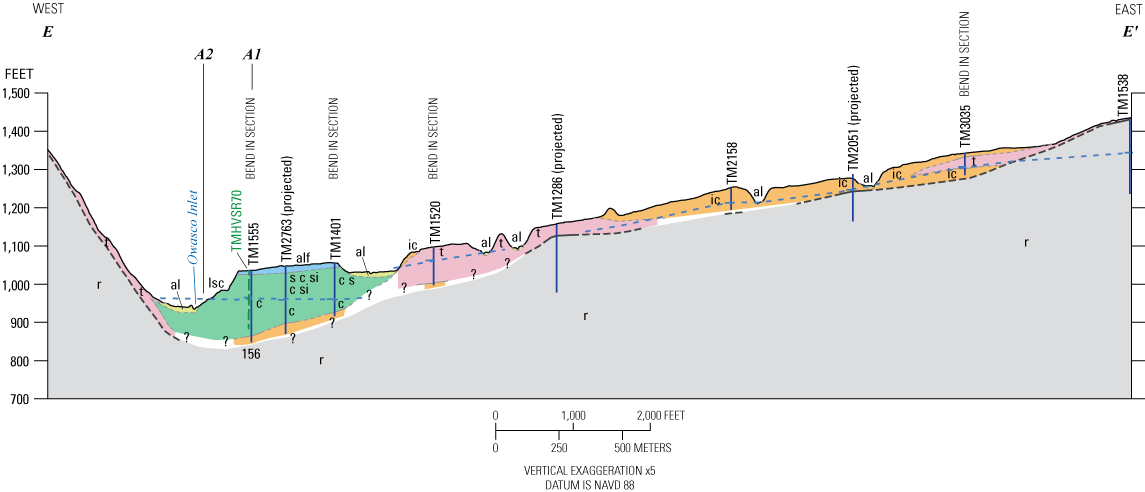

Hydrogeologic section E–E′ across the Owasco Inlet valley, about 1.3 miles (mi) north of Groton village, Tompkins County, New York. For location of cross section, wells, and other labeled features, see plate 1. HVSR, horizontal-to-vertical spectral ratio; NAVD, North American Vertical Datum of 1988; ND, no data.
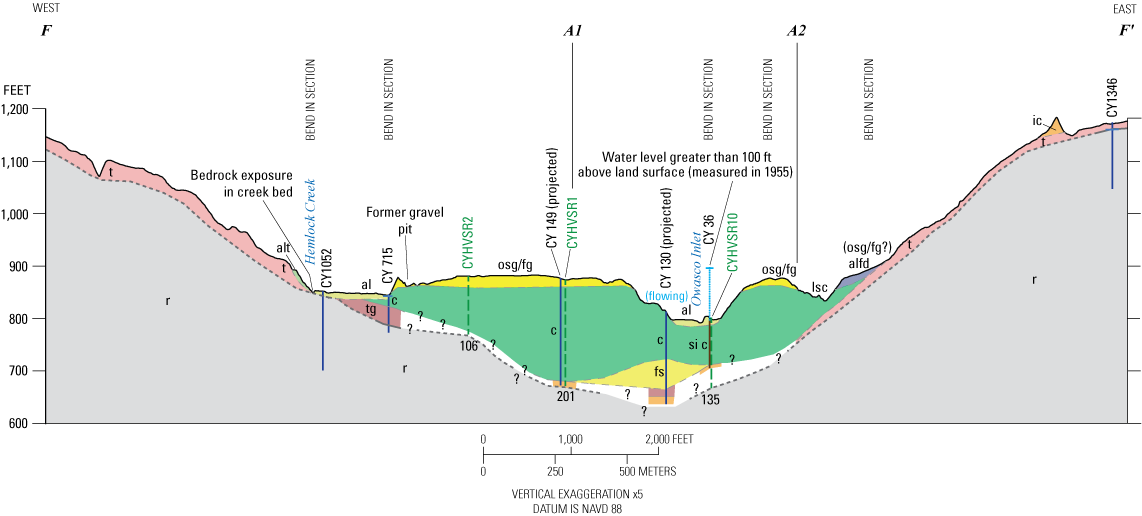

Hydrogeologic section F–F’ across the Owasco Inlet valley and Hemlock Creek valley at Locke, Cayuga County, New York. For location of cross section, wells, and other labeled features, see plate 1. HVSR, horizontal-to-vertical spectral ratio; NAVD, North American Vertical Datum of 1988.
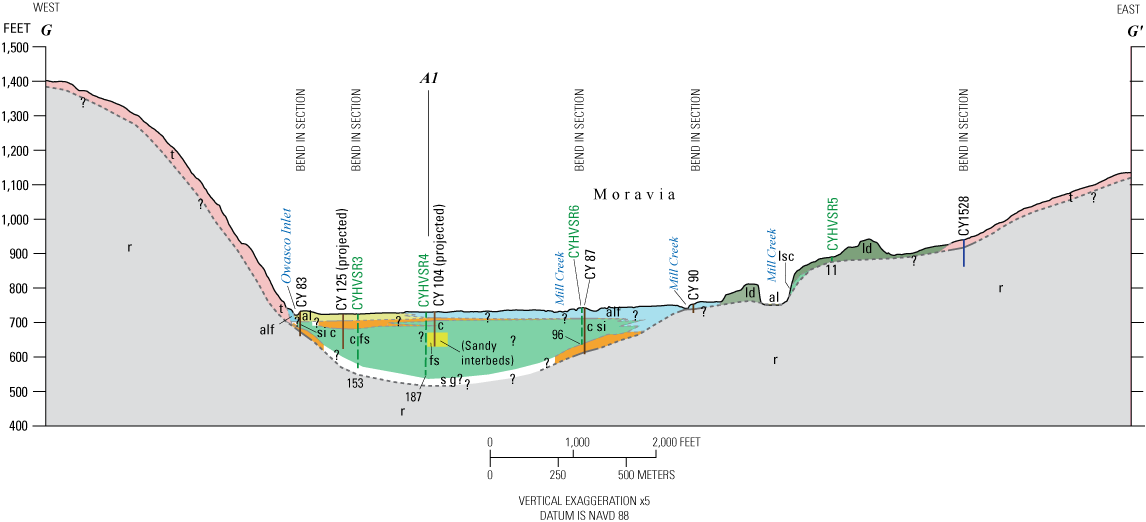

Hydrogeologic section G–G’ across the Owasco Inlet valley at Moravia, Cayuga County, New York. For location of cross section, wells, and other labeled features, see plate 1. HVSR, horizontal-to-vertical spectral ratio; NAVD, North American Vertical Datum of 1988.
References Cited
Bird, B.C., 2014, Proglacial lake reconstruction in Cayuga County, in Kozlowski, A. and Graham, B., eds., Glacial geology of Cayuga County of the eastern Finger Lakes—Lakes, lore, and landforms—North Eastern Friends of the Pleistocene Field Conference, 77th Annual Reunion, Auburn, N.Y., June 7–8, 2014, [Proceedings]: Albany, N.Y., New York State Geological Survey, New York State Museum/New York State Education Department, NYSGS Open File Report EZRA–14–06–01, p. 14–35. [Also available at https://www2.newpaltz.edu/fop/pdf/FOP2014Guide.pdf.]
Bird, B.C., and Kozlowski, A.L., 2014, Using LiDAR to reconstruct glacial lakes in Cayuga County, New York [abs.]: Geological Society of America Abstracts with Programs, v. 46, no. 2, p. 71. [Also available at https://gsa.confex.com/gsa/2014NE/webprogram/Paper236506.html.]
Bird, B.C., and Kozlowski, A.L., 2016a, Surficial geology of the Moravia 7.5 minute quadrangle—Cayuga and Tompkins Counties, New York: New York State Museum Map and Chart no. 89, 1 sheet, scale 1:24,000, accessed January 20, 2017, at https://www.nysm.nysed.gov/staff-publications/surficial-geology-moravia-quadrangle-cayuga-county-new-yo.
Bird, B.C., and Kozlowski, A.L., 2016b, Surficial geology of the Sempronius quadrangle, Cayuga County—New York: New York State Museum Map and Chart no. 90, 1 sheet, scale 1:24,000, accessed January 20, 2017, at https://www.nysm.nysed.gov/staff-publications/surficial-geology-sempronius-quadrangle-cayuga-county-new.
Carney, F., 1909, The Pleistocene geology of the Moravia quadrangle, New York: Bulletin of the Scientific Laboratories of Denison University [Granville, Ohio], v. XIV, p. 335–441, 1 pl., accessed January 19, 2017, at https://babel.hathitrust.org/cgi/pt?id=mdp.39015035473613&view=1up&seq=10.
Church, M., and Ryder, J.M., 1972, Paraglacial sedimentation—A consideration of fluvial processes conditioned by glaciation: Geological Society of America Bulletin, October 1972, v. 83, no. 10, p. 3059–3072, accessed January 18, 2017, at https://doi.org/10.1130/0016-7606(1972)83[3059:PSACOF]2.0.CO;2.
Clark, C.D., 1993, Mega-scale glacial lineations and cross-cutting ice-flow landforms: Earth Surface Processes and Landforms, v. 18, no. 1, p. 1–29, accessed January 17, 2017, at https://doi.org/10.1002/esp.3290180102.
Corbett, L.B., Bierman, P.R., Stone, B.D., Caffee, M.W., and Larsen, P.L., 2017, Cosmogenic nuclide age estimate for Laurentide Ice Sheet recession from the terminal moraine, New Jersey, USA, and constraints on latest Pleistocene ice sheet history: Quaternary Research, v. 87, no. 3, p. 482–498, accessed February 13, 2018, at https://doi.org/10.1017/qua.2017.11.
Denny, C.S., and Lyford, W.H., 1963, Surficial geology and soils of the Elmira-Williamsport region, New York and Pennsylvania: U.S. Geological Survey Professional Paper 379, 59 p., 6 pls., accessed January 17, 2017, at https://doi.org/10.3133/pp379.
Dyke, A.S., 2004, An outline of North American deglaciation with emphasis on central and northern Canada, in Part B of Ehlers, J., and Gibbard, P.L., eds., Quaternary glaciations, Extent and chronology: Developments in Quaternary Science, v. 2, p. 373–424, accessed January 17, 2017, at https://doi.org/10.1016/S1571-0866(04)80209-4.
Fairchild, H.L., 1932, New York moraines: Geological Society of America Bulletin, v. 43, no. 3, p. 627–662, accessed January 11, 2017, at https://doi.org/10.1130/GSAB-43-627.
Fairchild, H.L., 1934, Cayuga valley lake history: Geological Society of America Bulletin, v. 45, no. 2, p. 233–280, accessed January 11, 2017, at https://doi.org/10.1130/GSAB-45-233.
Heisig, P.M., 1999, Water resources of the Batavia Kill basin at Windham, Greene County, New York: U.S. Geological Survey Water-Resources Investigations Report 1998–4036, 96 p., accessed January 11, 2016, at https://pubs.er.usgs.gov/publication/wri984036. [Also available at https://doi.org/10.3133/wri984036.]
Heisig, P.M., 2023a, Geospatial datasets of the glacial geology and hydrogeology of the Owasco Inlet Watershed, Cayuga and Tompkins Counties, New York: U.S. Geological Survey data release, https://doi.org/10.5066/P9E6ESC2.
Heisig, P.M., 2023b, Horizontal-to-vertical spectral ratio (HVSR) soundings and depth-to-bedrock data for the Owasco Inlet watershed, Cayuga and Tompkins Counties, New York 2016: U.S. Geological Survey data release, https://doi.org/10.5066/P9GI61NV.
Heisig, P.M. and Fleisher, P.J., 2022, Glacial geology and hydrogeology of valley-fill aquifers in the Oneonta area, Otsego and Delaware Counties, New York: U.S. Geological Survey Scientific Investigations Report 2022–5069, 35 p., accessed August 19, 2022, at https://doi.org/10.3133/sir20225069.
Karig, D.E., 2015, Quaternary geology of the greater Sixmile Creek watershed: Cornell University Library eCommons, technical report, 66 p., 6 pl., 2 app., accessed May 6, 2016, at https://hdl.handle.net/1813/40572.
Karig, D.E., 2022, A partial geologic history of Fall Creek, Tompkins County, New York: Cornell University Library eCommons, technical report, 41 p., accessed July 25, 2022, at https://ecommons.cornell.edu/handle/1813/111371.
Karig, D.E., and Isacks, B.L., 2019, A revised history of late Quaternary glaciation in the Cayuga basin, trip A1 a in Kendrick, D.C., ed., New York State Geological Association Field Trip Guidebook, 91st Annual Meeting, Geneva, N.Y., October 5–6, 2019: [New York State Council of Professional Geologists and Hobart and William Smith Colleges], p. 9–46. [Also available at https://www.nysga-online.org/guidebooks/by-year/.]
Karig, D.E., and Miller, T.S., 2020, Northward subglacial drainage during the Mackinaw Interstade in the Cayuga basin, central New York, USA: Canadian Journal of Earth Sciences, v. 57, no. 8, p. 981–998, accessed December 11, 2020, at https://doi.org/10.1139/cjes-2019-0111.
Kozlowski, A., and Graham, B., eds., 2014, Glacial geology of Cayuga County of the eastern Finger Lakes—Lakes, lore, and landforms—North Eastern Friends of the Pleistocene Field Conference, 77th Annual Reunion, Auburn, N.Y., June 7–8, 2014 [Proceedings], Albany, N.Y., New York State Geological Survey, New York State Museum/New York State Education Department, NYSGS Open File Report EZRA–14–06–01, 133 p., accessed January 11, 2017, at https://www2.newpaltz.edu/fop/pdf/FOP2014Guide.pdf.
Lane, J.W., Jr., White, E.A., Steele, G.V., and Cannia, J.C., 2008, Estimation of bedrock depth using the horizontal-to-vertical (H/V) ambient-noise seismic method, in Volume 2 of 21st Symposium on the application of geophysics to engineering and environmental problems 2008, Philadelphia, April 6–10, 2008, [Proceedings]: Denver, Colo., Environmental and Engineering Geophysical Society, p. 490–502, accessed January 8, 2018, at https://doi.org/10.4133/1.2963289.
Lewis, C.F.M., Blasco, S.M., and Gareau, P.L., 2005, Glacial isostatic adjustment of the Laurentian Great Lakes Basin—Using the empirical record of strandline deformation for reconstruction of early Holocene paleo-lakes and discovery of a hydrologically closed phase: Géographie Physique et Quaternaire, v. 59, no. 2–3, p. 187–210, accessed January 8, 2018, at https://doi.org/10.7202/014754ar.
Morrissey, D.J., Randall, A.D., and Williams, J.H., 1988, Upland runoff as a major source of recharge to stratified drift in the glaciated northeast, in Randall, A.D., and Johnson, A.I., eds., Regional aquifer systems of the United States, The northeast glacial aquifers, AWRA symposium on monitoring, modeling, and mediating water quality, Syracuse, N.Y., May 17–20, 1987: American Water Resources Association Monograph Series no. 11, p. 17–36.
Muller, E.H., and Calkin, P.E., 1993, Timing of Pleistocene glacial events in New York State: Canadian Journal of Earth Sciences, v. 30, no. 9, p. 1829–1845, accessed January 8, 2018, at https://doi.org/10.1139/e93-161.
Muller, E.H., and Prest, V.K., 1985, Glacial lakes in the Ontario basin, in Karrow, P.F., and Calkin, P.E., eds., Quaternary evolution of the Great Lakes: Geological Association of Canada Special Paper 30, p. 213–229, accessed January 8, 2018, at https://gac.ca/product/quaternary-evolution-of-the-great-lakes/.
Mullins, H.T., Hinchey, E.J., Wellner, R.W., Stephens, D.B., Anderson, W.T., Jr., Dwyer, T.R., and Hine, A.C., 1996, Seismic stratigraphy of the Finger Lakes—A continental record of Heinrich event H–1 and Laurentide ice sheet instability, in Mullins, H.T., and Eyles, N., eds., Subsurface geologic investigations of New York Finger Lakes—Implications for late Quaternary deglaciation and environmental change: Boulder, Colo., Geological Society of America Special Paper 311, p. 1–35, accessed January 8, 2018, at https://doi.org/10.1130/0-8137-2311-6.1]
Randall, A.D., 1978, Infiltration from tributary streams in the Susquehanna River Basin: Journal of Research of the U.S. Geological Survey, v. 6, no. 3, p. 285–297, 1 pl., scale 1:24,000, accessed January 8, 2018, at https://doi.org/10.3133/70007426.
Randall, A.D., 2001, Hydrogeologic framework of stratified-drift aquifers in the glaciated northeastern United States: U.S. Geological Survey Professional Paper 1415–B, 179 p., 1 pl., accessed January 8, 2018, at https://doi.org/10.3133/pp1415B.
Rickard, L.V., and Fisher, D.W., 1970, Geologic map of New York—Finger Lakes sheet: New York State Museum and Science Service Map and Chart Series no. 15, 1 sheet, scale 1:250,000, accessed January 8, 2018, at https://www.nysm.nysed.gov/research-collections/geology/gis.
Ridge, J.C., 2003, The last deglaciation of the northeastern United States—A combined varve, paleomagnetic, and calibrated 14C chronology, chap. 3 of Cremeens, D.L., and Hart, J.P., eds., Geoarcheology of landscapes in the glaciated Northeast: Albany, N.Y, New York State Education Department, New York State Museum Bulletin 497, p. 15–45, accessed January 8, 2018, at https://www.nysm.nysed.gov/publications/bulletins.
Stokes, C.R., 2017, Deglaciation of the Laurentide ice sheet from the last glacial maximum: Cuadernos de Investigación Geográfica, v. 43, no. 2, p. 377–428, accessed January 8, 2018, at https://doi.org/10.18172/cig.3237.
U.S. Department of Agriculture [USDA], 2008, Soil survey geographic (SSURGO) database for Cayuga and Tompkins Counties, New York: Natural Resources Conservation Service, U.S. Department of Agriculture database, accessed February 9, 2016, at http://soildatamart.nrcs.usda.gov/. [Also available at https://websoilsurvey.sc.egov.usda.gov/App/HomePage.htm.]
U.S. Environmental Protection Agency, 2012, 2012 edition of the drinking water standards and health advisories: Washington, D.C., U.S. Environmental Protection Agency, Office of Water, EPA 822–S–12–001, 12 p., accessed April 3, 2023, at https://hero.epa.gov/hero/index.cfm/reference/details/reference_id/1936016.
U.S. Environmental Protection Agency [EPA], [2017], Safe drinking water information system (SDWIS) Federal reporting services system: U.S. Environmental Protection Agency database, accessed July 14, 2017, at https://oaspub.epa.gov/enviro/sdw_form_v2.create_page?state_abbr=NY. [Also available at https://ordspub.epa.gov/ords/sfdw/sfdw/r/sdwis_fed_reports_public/200.]
U.S. Geological Survey, 2022, USGS water data for the Nation: U.S. Geological Survey National Water Information System database, accessed May 11, 2022, at https://doi.org/10.5066/F7P55KJN. [Site information directly accessible at https://nwis.waterdata.usgs.gov/usa/nwis/qwdata.]
Walter, D.A., Masterson, J.P., Finkelstein, J.S., Monti, J., Jr., Misut, P.E., and Fienen, M.N., 2020, Simulation of groundwater flow in the regional aquifer system on Long Island, New York, for pumping and recharge conditions in 2005–15: U.S. Geological Survey Scientific Investigations Report 2020–5091, 75 p., accessed January 20, 2021, at https://doi.org/10.3133/sir20205091.
Williams, H.S., Tarr, R.S., and Kindle, E.M., 1909, Watkins Glen-Catatonk folio, New York: Folios of the Geologic Atlas 169, 33 p., 6 pls. [Also available at https://doi.org/10.3133/gf169.]
Conversion Factors
U.S. customary units to International System of Units
Temperature in degrees Celsius (°C) may be converted to degrees Fahrenheit (°F) as follows:
°F = (1.8 × °C) + 32.
Temperature in degrees Fahrenheit (°F) may be converted to degrees Celsius (°C) as follows:
°C = (°F – 32) / 1.8.
Datum
Vertical coordinate information is referenced to the North American Vertical Datum of 1988 (NAVD 88).
Horizontal coordinate information is referenced to the North American Datum of 1983 (NAD 83).
Elevation, as used in this report, refers to distance above the vertical datum.
Supplemental Information
Specific conductance is given in microsiemens per centimeter at 25 degrees Celsius (µS/cm at 25 °C).
Concentrations of chemical constituents in water are given in either milligrams per liter (mg/L) or micrograms per liter (µg/L).
B.P., before present (dates before 1950, in thousands of years).
Abbreviations
EPA
U.S. Environmental Protection Agency
HVSR
horizontal-to-vertical spectral ratio
lidar
light detecting and ranging
NWIS
National Water Information System (USGS)
NYSDEC
New York State Department of Environmental Conservation
NYSGS
New York State Geological Survey
SSURGO
Soil-Survey Geographic database (USDA)
USDA
U.S. Department of Agriculture
USGS
U.S. Geological Survey
For more information about this report, contact:
Director, New York Water Science Center
U.S. Geological Survey
425 Jordan Road
Troy, NY 12180–8349
dc_ny@usgs.gov
(518) 285–5602
or visit our website at
Suggested Citation
Heisig, P.M., 2023, Hydrogeology of sand and gravel aquifers in the Owasco Inlet watershed, Cayuga and Tompkins Counties, New York: U.S. Geological Survey Scientific Investigations Report 2023–5031, 32 p., 1 pl., https://doi.org/10.3133/sir20235031.
ISSN: 2328-0328 (online)
Study Area
Publication type | Report |
---|---|
Publication Subtype | USGS Numbered Series |
Title | Hydrogeology of sand and gravel aquifers in the Owasco Inlet watershed, Cayuga and Tompkins Counties, New York |
Series title | Scientific Investigations Report |
Series number | 2023-5031 |
DOI | 10.3133/sir20235031 |
Year Published | 2023 |
Language | English |
Publisher | U.S. Geological Survey |
Publisher location | Reston, VA |
Contributing office(s) | New York Water Science Center |
Description | Report: vii, 32 p.; 2 Data Releases; 1 Plate: 24.00 × 36.00 inches |
Country | United States |
State | New York |
County | Cayuga County, Tompkins County |
Other Geospatial | Owasco Inlet Watershed |
Online Only (Y/N) | Y |
Additional Online Files (Y/N) | Y |
Google Analytic Metrics | Metrics page |